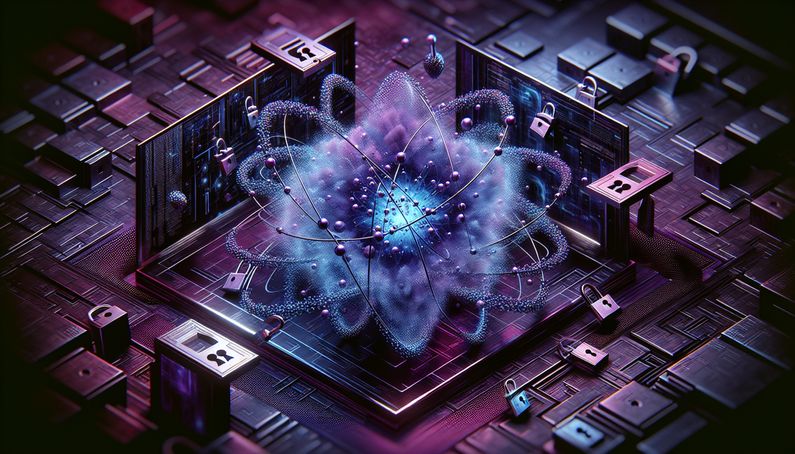
The Impact of Quantum Computing on Information Security
Quantum computing represents a paradigm shift in computational capabilities, leveraging the principles of quantum mechanics to perform calculations at speeds unattainable by classical computers. Unlike traditional systems that use binary bits, quantum computers utilize qubits, which can exist in multiple states simultaneously, thanks to phenomena like superposition and entanglement (Quantum Computing Inc). This unique capability allows quantum computers to tackle complex problems, such as integer factorization, exponentially faster than their classical counterparts, posing both opportunities and challenges for information security.
The potential of quantum computing to disrupt current cryptographic systems is particularly concerning. Algorithms like RSA and ECC, which underpin much of today’s secure communications, could be rendered obsolete by quantum algorithms such as Shor’s algorithm, capable of breaking these encryptions in a fraction of the time required by classical methods (KPMG Australia). This looming threat has spurred the development of post-quantum cryptography (PQC), with organizations like the National Institute of Standards and Technology (NIST) leading efforts to standardize quantum-resistant algorithms (CISA, 2024).
As we stand on the brink of the quantum era, the implications for information security are profound. The transition to quantum-safe systems is fraught with challenges, including interoperability with legacy systems, resource constraints, and the complexity of implementation (GovLoop). However, the potential benefits, such as enhanced cryptographic methods and real-time threat detection, offer a glimpse into a future where quantum computing not only poses risks but also provides robust solutions to emerging cybersecurity challenges.
Quantum Computing: A Brief Overview
The Fundamentals of Quantum Computing
Quantum computing operates on the principles of quantum mechanics, a branch of physics that describes the behavior of particles at the atomic and subatomic levels. Unlike classical computers, which process information in binary bits (0s and 1s), quantum computers use quantum bits, or qubits. Qubits can exist in a superposition of states, meaning they can represent both 0 and 1 simultaneously. This property enables quantum computers to perform complex calculations at speeds unattainable by classical systems.
Entanglement, another quantum phenomenon, allows qubits to be interconnected such that the state of one qubit instantly influences the state of another, regardless of distance. This interconnectedness enhances computational power exponentially. (Quantum Computing Inc).
Key Components of Quantum Computing
Qubits and Their Role
Qubits are the fundamental units of quantum information. They are implemented using various physical systems, such as photons, trapped ions, and superconducting circuits. Unlike classical bits, qubits leverage quantum properties like superposition and entanglement to perform parallel computations. This capability allows quantum computers to solve problems that are infeasible for classical computers.
For example, a quantum computer with 300 qubits could theoretically perform calculations on 2^300 possible states simultaneously, a number far exceeding the total atoms in the observable universe. (TechTarget).
Quantum Gates and Circuits
Quantum gates manipulate qubits and are the building blocks of quantum algorithms. These gates operate differently from classical logic gates, as they perform operations on qubits in superposition. Quantum circuits are networks of quantum gates designed to solve specific computational problems. For instance, the Shor’s algorithm, a quantum algorithm for integer factorization, uses quantum gates to solve problems exponentially faster than classical algorithms. (SpringerLink).
Quantum Decoherence and Error Correction
Quantum decoherence is a significant challenge in quantum computing. It occurs when qubits lose their quantum state due to interaction with the environment, leading to errors in computation. Quantum error correction techniques, such as surface codes and topological codes, are being developed to mitigate these issues. These methods involve encoding quantum information redundantly across multiple qubits to detect and correct errors. (AzoQuantum).
Current Applications of Quantum Computing
Cryptography and Security
One of the most discussed applications of quantum computing is its impact on cryptography. Quantum computers have the potential to break widely-used encryption methods, such as RSA and ECC, by efficiently solving mathematical problems like integer factorization and discrete logarithms. This capability poses a significant threat to data security.
To counteract these risks, researchers are developing post-quantum cryptographic algorithms that are resistant to quantum attacks. The National Institute of Standards and Technology (NIST) is leading efforts to standardize these algorithms. (Analytics Insight).
Optimization Problems
Quantum computing excels in solving optimization problems, which involve finding the best solution from a set of possible solutions. Applications include supply chain optimization, financial portfolio management, and traffic flow optimization. For example, quantum algorithms can optimize delivery routes for logistics companies, reducing costs and improving efficiency. (The Silicon Review).
Drug Discovery and Material Science
Quantum computers can simulate molecular interactions at an unprecedented level of detail, enabling breakthroughs in drug discovery and material science. For instance, they can model complex proteins to identify potential drug candidates or design new materials with desired properties. This capability has the potential to revolutionize industries such as pharmaceuticals and manufacturing. (LevelBlue).
Challenges in Quantum Computing Development
Scalability and Qubit Stability
Building scalable quantum computers with stable qubits remains a significant challenge. Current quantum systems are limited in the number of qubits they can reliably maintain. Achieving fault-tolerant quantum computing requires advancements in qubit design, error correction, and quantum interconnects. (TechTarget).
High Costs and Resource Requirements
Quantum computing research and development require substantial financial and technical resources. Building and maintaining quantum hardware involves sophisticated infrastructure, such as cryogenic systems to maintain qubits at near-absolute zero temperatures. These costs limit access to quantum computing technology, making it primarily available to large organizations and research institutions. (TimesPro).
Ethical and Security Concerns
The potential misuse of quantum computing for malicious purposes, such as breaking encryption or developing advanced cyberattacks, raises ethical and security concerns. Establishing international regulations and ethical guidelines is crucial to ensure the responsible development and use of quantum technology. (Hivenet).
Future Prospects of Quantum Computing
Quantum Supremacy and Beyond
Quantum supremacy, the point at which quantum computers outperform classical computers in specific tasks, has been achieved in controlled experiments. However, transitioning from experimental demonstrations to practical applications requires overcoming technical and operational challenges. The integration of quantum computing with classical systems is expected to unlock new possibilities in fields such as artificial intelligence and big data analytics. (The Quantum Insider).
Global Collaboration and Standardization
International collaboration is essential to address the challenges and opportunities of quantum computing. Organizations like NIST and the European Telecommunications Standards Institute (ETSI) are working on standardizing quantum-resistant cryptographic algorithms and protocols. These efforts aim to ensure the global adoption of secure quantum technologies. (AzoQuantum).
Integration with Emerging Technologies
Quantum computing is expected to complement other emerging technologies, such as artificial intelligence, blockchain, and the Internet of Things (IoT). For instance, quantum algorithms can enhance machine learning models by solving optimization problems more efficiently. Similarly, quantum-safe blockchain protocols can ensure secure transactions in a quantum-enabled world. (Analytics Insight).
By focusing on these aspects, the report provides a comprehensive overview of quantum computing and its implications for information security, addressing gaps not covered in existing reports.
The Threat to Information Security
Quantum Computing and Cryptographic Vulnerabilities
Quantum computing poses a significant threat to traditional cryptographic systems, especially those using public-key cryptography. Algorithms like RSA, ECC (Elliptic Curve Cryptography), and DSA (Digital Signature Algorithm) rely on complex mathematical problems that are difficult for classical computers to solve. However, quantum algorithms such as Shor’s algorithm can solve these problems much faster, potentially making these cryptographic methods ineffective.
For example, RSA-2048, a common encryption standard, would take classical supercomputers thousands of years to break. In contrast, a powerful quantum computer could decrypt it in just hours (KPMG Australia). This has led to the urgent development of post-quantum cryptography (PQC), which aims to create algorithms resistant to quantum attacks. The National Institute of Standards and Technology (NIST) is actively working on standardizing quantum-resistant cryptographic algorithms, with final recommendations expected by 2025.
”Store Now, Decrypt Later” Attacks
A growing concern in cybersecurity is the “store now, decrypt later” strategy used by malicious actors. This involves intercepting and storing encrypted data today with the intent of decrypting it in the future when quantum computing capabilities become available. Sensitive information such as financial records, government communications, and personal data could be at risk.
Adversaries are already collecting encrypted data in anticipation of future quantum decryption capabilities (The Soufan Center). This threat highlights the need for organizations to transition to quantum-safe cryptographic systems proactively. The timeline for this transition is critical, as encrypted data often has a long shelf life, and failure to act could result in significant breaches in the coming years.
Threats to Critical Infrastructure
Quantum computing’s ability to break encryption poses a direct threat to critical infrastructure systems, including energy grids, transportation networks, and healthcare systems. Many of these systems rely on legacy cryptographic protocols that are vulnerable to quantum attacks. A successful breach could lead to catastrophic consequences, such as power outages, compromised medical devices, or disrupted public services.
The urgency of addressing these vulnerabilities is highlighted in reports such as the Quantum Threat Timeline Report, which emphasizes that organizations must begin implementing quantum-safe cryptography immediately. The report also notes that the global competition to advance quantum computing capabilities is accelerating, increasing the likelihood of these threats materializing sooner than anticipated.
Implications for Data Privacy
The advent of quantum computing could have profound implications for data privacy. Personal information, financial data, and intellectual property stored in encrypted formats could become accessible to unauthorized parties. This is particularly concerning in industries such as finance, healthcare, and technology, where data breaches could have severe consequences.
A study by Gartner revealed that 70% of Chief Information Security Officers (CISOs) plan to implement quantum-safe cryptography by 2025 to safeguard sensitive information (Vorecol). However, the transition to quantum-resistant systems is complex and resource-intensive, requiring significant investment in technology and training.
Quantum-Safe Networks and Solutions
To mitigate the risks posed by quantum computing, organizations are exploring quantum-safe networks and technologies. These solutions integrate quantum-resistant cryptographic algorithms and quantum key distribution (QKD) to enhance data security. QKD uses the principles of quantum mechanics to create encryption keys that are theoretically immune to interception.
For example, Nokia has developed quantum-safe networks designed to protect against future cyber threats. These networks aim to prevent attacks where encrypted data is harvested and stored for future decryption. By adopting such technologies, organizations can ensure their data remains secure in the quantum era.
Challenges in Transitioning to Quantum-Resistant Systems
While the need for quantum-resistant systems is clear, the transition poses several challenges. These include scalability, cost, and the complexity of integrating new algorithms into existing systems. Additionally, organizations must inventory their current cryptographic measures and assess their vulnerabilities to quantum threats.
The Global Risk Institute emphasizes that implementing quantum-safe cryptography requires time and resources to ensure success. Errors in this process could lead to vulnerabilities, making it essential for organizations to adopt a well-planned approach. Training and awareness programs for senior leadership are also critical to ensure a smooth transition.
Emerging Quantum Threats in National Security
Quantum computing’s potential to break encryption poses a significant risk to national security. Encrypted communications, defense systems, and intelligence data could become vulnerable to quantum attacks. Governments worldwide are investing in quantum research to stay ahead of these threats and develop quantum-resistant solutions.
For instance, the Belfer Center highlights the importance of transitioning to quantum-resistant cryptographic approaches to protect classified information. The center also notes that the timeline for quantum computing breakthroughs aligns closely with the time required to implement these new systems, making immediate action imperative.
Quantum Computing and Cybercrime
The rise of quantum computing could also lead to more sophisticated cybercrime. Malicious actors could use quantum computers to enhance their offensive capabilities, such as cracking passwords, bypassing authentication systems, and launching more effective phishing attacks. This would make it increasingly difficult for organizations to defend against cyber threats.
Reports such as ESET emphasize the need for robust defense mechanisms to counter these evolving threats. Organizations must invest in advanced threat detection and response systems to stay ahead of cybercriminals in the quantum era.
The Role of International Collaboration
Addressing the threats posed by quantum computing requires international collaboration. Governments, private organizations, and research institutions must work together to develop and standardize quantum-resistant technologies. Initiatives such as the NIST post-quantum cryptography project and the European Quantum Flagship program are examples of efforts to tackle these challenges on a global scale.
The Soufan Center highlights the importance of global cooperation in mitigating quantum threats. By sharing knowledge and resources, stakeholders can accelerate the development of quantum-safe solutions and ensure a coordinated response to emerging risks.
Preparing for the Quantum Era
Organizations must take proactive steps to prepare for the quantum era. This includes conducting risk assessments, developing contingency plans, and investing in quantum-resistant technologies. Training programs for employees and leadership are also essential to build awareness and ensure a smooth transition.
The KPMG Australia report outlines key actions for mitigating quantum risks, such as modernizing cryptographic environments and providing guidance on quantum-resistant technologies. By taking these steps, organizations can safeguard their information security in the face of quantum threats.
Preparing for the Quantum Era
Understanding the Quantum Threat Landscape
The advent of quantum computing presents a significant challenge to current cryptographic systems, which rely on the computational difficulty of problems like integer factorization and discrete logarithms. Quantum computers, leveraging algorithms such as Shor’s algorithm, could potentially break widely used encryption methods like RSA and ECC within a matter of seconds. This has created urgency among organizations to evaluate their cryptographic infrastructure and prepare for a quantum-secure future. Reports indicate that quantum computers capable of breaking these cryptographic systems could emerge within the next 10 to 15 years (Global Risk Institute, 2024).
Developing Quantum-Safe Cryptographic Standards
Efforts to establish quantum-resistant cryptographic standards are being led by organizations like the National Institute of Standards and Technology (NIST). NIST has been working on standardizing post-quantum cryptographic (PQC) algorithms since 2016, with the first set of draft standards expected to be finalized by 2024 (CISA, 2024). These algorithms are designed to resist attacks from both classical and quantum computers, ensuring long-term data security.
Key steps in transitioning to PQC include:
- Inventorying Cryptographic Assets: Organizations must identify and categorize all systems and data relying on cryptographic protections. This includes assessing the sensitivity and lifespan of the data (Forbes, 2024).
- Testing and Piloting: Early testing of NIST-approved algorithms within organizational infrastructure is crucial. Pilot programs can help identify integration challenges and refine implementation strategies (SC Media, 2024).
- Collaboration with Vendors: Organizations must work closely with technology vendors to ensure their products are updated to support PQC algorithms (CISA, 2024).
Building Crypto-Agility into Systems
Crypto-agility refers to the ability of a system to quickly adapt to new cryptographic algorithms without significant disruption. This capability is critical for ensuring a smooth transition to post-quantum cryptography. Organizations should design systems that allow for seamless swapping of cryptographic algorithms as standards evolve. This involves:
- Modular Cryptographic Architectures: Implementing modular frameworks that enable easy integration of new algorithms (EY, 2024).
- Regular Updates and Patching: Ensuring systems are regularly updated to incorporate the latest cryptographic advancements.
- Training and Awareness: Educating IT teams on the principles of crypto-agility and the importance of maintaining flexibility in cryptographic systems.
Assessing Organizational Readiness
Organizations must evaluate their preparedness for the quantum era by conducting comprehensive risk assessments. This includes:
- Evaluating Data Sensitivity: Identifying data that could be at risk of future quantum attacks, particularly information with long-term sensitivity (Global Risk Institute, 2024).
- Understanding the Threat Timeline: Staying informed about advancements in quantum computing and the projected timeline for quantum threats (CIS Security, 2024).
- Developing a Transition Plan: Creating a detailed roadmap for adopting PQC, including timelines, resource allocation, and integration strategies (SC Media, 2024).
Regulatory and Industry Collaboration
Governments and industry bodies are playing a crucial role in driving the transition to quantum-safe cryptography. In the United States, the Quantum Computing Cybersecurity Preparedness Act mandates federal agencies to begin transitioning to quantum-resistant cryptographic systems (CISA, 2024). Similarly, international collaborations are fostering the development of best practices and standards for quantum security.
Organizations should:
- Engage with Regulatory Bodies: Stay updated on regulatory requirements and participate in standardization efforts.
- Collaborate Across Industries: Share insights and strategies with peers to address common challenges in transitioning to PQC (The Digital Speaker, 2024).
Investing in Research and Development
To stay ahead of the quantum threat, organizations must invest in research and development (R&D) focused on quantum-resistant technologies. This includes:
- Exploring Emerging Solutions: Investigating novel cryptographic approaches, such as lattice-based cryptography and hash-based signatures.
- Partnering with Academia and Startups: Collaborating with research institutions and innovative startups to accelerate the development of quantum-safe solutions (Global Risk Institute, 2024).
- Monitoring Technological Advancements: Keeping track of breakthroughs in quantum computing and their implications for cybersecurity.
By proactively addressing these areas, organizations can mitigate the risks posed by quantum computing and ensure the security of their information systems in the quantum era.
Challenges in Transitioning to Quantum-Safe Security
Interoperability with Legacy Systems
One of the most significant challenges in transitioning to quantum-safe security is ensuring interoperability between new quantum-resistant algorithms and existing legacy systems. Many organisations rely on infrastructure that has been in place for decades, and replacing or upgrading these systems to support quantum-safe cryptography (QSC) is a complex task. Legacy systems are often tightly integrated into operational workflows, making it difficult to implement new cryptographic standards without disrupting business processes.
For example, transitioning to quantum-safe Transport Layer Security (TLS) protocols requires updating not only the cryptographic libraries but also the applications, middleware, and hardware that rely on these protocols. This process is further complicated by the lack of universal standards for quantum-safe algorithms, which are still being developed and tested. (GovLoop)
Resource Constraints and Cost Implications
The financial and resource burden of transitioning to quantum-safe security is another major hurdle. Organisations must allocate significant budgets for upgrading infrastructure, training personnel, and conducting comprehensive risk assessments. According to industry estimates, the cost of transitioning to post-quantum cryptography (PQC) could run into billions of dollars globally, as organisations scramble to replace vulnerable cryptographic systems before quantum computers become a practical threat.
Additionally, the workforce required to implement these changes must be adequately trained in quantum-safe technologies. This includes not only IT professionals but also cybersecurity teams, who must understand the nuances of quantum-resistant algorithms and their implications for organisational security. The lack of skilled professionals in this niche field further exacerbates the challenge. (IBM)
Performance Trade-offs
Quantum-safe algorithms often require more computational resources than traditional cryptographic methods, leading to potential performance trade-offs. For example, lattice-based cryptographic algorithms, which are among the most promising candidates for post-quantum cryptography, can be computationally intensive. This increased resource demand can result in slower processing times for encryption and decryption, which may not be acceptable for high-performance applications such as financial transactions or real-time communications.
Moreover, the larger key sizes and signatures associated with quantum-safe algorithms can lead to increased storage and bandwidth requirements. This is particularly problematic for resource-constrained environments, such as Internet of Things (IoT) devices, which may not have the capacity to handle these additional demands. (SSL.com)
Uncertainty in Quantum Computing Timelines
The uncertainty surrounding the timeline for the development of cryptographically relevant quantum computers adds another layer of complexity to the transition. While some experts predict that such computers could be operational within the next 5-10 years, others believe it may take longer. This uncertainty makes it difficult for organisations to prioritise and plan their transition to quantum-safe security.
For instance, organisations must decide whether to invest in immediate upgrades or wait for more mature quantum-safe standards to emerge. This dilemma is compounded by the rapid pace of advancements in quantum computing, which could render current quantum-safe solutions obsolete before they are fully implemented. (Forbes)
Complexity of Implementation
Implementing quantum-safe cryptography is not a straightforward process. It requires a comprehensive overhaul of existing cryptographic infrastructures, including protocols, standards, and libraries. This complexity is further amplified by the need to ensure compatibility across a diverse range of systems and applications. For example, hybrid cryptographic systems that integrate both classical and quantum-safe algorithms must be rigorously tested to detect and address potential interoperability issues.
Additionally, the standardisation process for quantum-safe algorithms is still ongoing, with organisations like the National Institute of Standards and Technology (NIST) leading the charge. Until these standards are finalised, organisations face the risk of investing in solutions that may not align with future standards. (Adacom)
Security Risks During Transition
The transition to quantum-safe security itself introduces new security risks. For instance, the process of replacing existing cryptographic protocols with quantum-resistant alternatives can create temporary vulnerabilities that cybercriminals may exploit. Additionally, the lack of widespread adoption of quantum-safe algorithms means that organisations may have to rely on hybrid systems, which combine classical and quantum-safe cryptography. These hybrid systems can be more complex to manage and may introduce new attack vectors.
Furthermore, the migration process often involves the use of automated tools to identify and replace vulnerable cryptographic systems. While these tools can expedite the transition, they are not foolproof and may overlook critical vulnerabilities or misconfigure new systems. This underscores the importance of thorough testing and validation during the transition phase. (CSO Online)
Lack of Awareness and Preparedness
A significant number of organisations are still unaware of the impending quantum threat or the steps they need to take to mitigate it. According to a recent survey, 68% of IT professionals are concerned that quantum computing will render their current encryption methods obsolete within the next five years. However, many organisations have yet to begin planning for the transition to quantum-safe security.
This lack of awareness is particularly prevalent among small and medium-sized enterprises (SMEs), which often lack the resources and expertise to address complex cybersecurity challenges. As a result, these organisations are at a higher risk of being targeted by cybercriminals once quantum computers become capable of breaking traditional encryption. (Medium)
Regulatory and Compliance Challenges
The regulatory landscape for quantum-safe security is still evolving, creating additional challenges for organisations. While some governments have issued guidelines and mandates for transitioning to quantum-safe cryptography, others have yet to address the issue. This lack of uniformity can make it difficult for multinational organisations to develop a cohesive strategy for adopting quantum-safe security.
Moreover, compliance with emerging quantum-safe standards may require organisations to undergo extensive audits and certifications, which can be both time-consuming and costly. For example, organisations operating in highly regulated industries, such as finance and healthcare, may face stricter requirements for implementing quantum-safe cryptography. (Government Executive)
Conclusion
The transition to quantum-safe security is a multifaceted challenge that requires careful planning, significant investment, and collaboration across the cybersecurity community. By addressing these challenges proactively, organisations can position themselves to navigate the quantum era successfully and ensure the continued security of their digital assets.
Opportunities in the Quantum Era
Quantum-Enhanced Cryptography
Quantum computing introduces opportunities to revolutionize cryptography by leveraging quantum mechanics to create encryption methods that are inherently more secure than classical approaches. Unlike classical encryption, which relies on mathematical complexity, quantum cryptography utilizes the principles of quantum mechanics, such as superposition and entanglement, to ensure data security. For instance, Quantum Key Distribution (QKD), a method that uses quantum bits (qubits) to generate encryption keys, ensures that any interception attempt disrupts the quantum state, alerting the parties involved. This feature makes QKD a promising candidate for securing sensitive communications in industries like finance, healthcare, and defense.
According to a recent report from a credible cybersecurity research firm, the adoption of QKD is projected to grow significantly between 2025 and 2030, with companies like IBM and Google leading the development of quantum-secure communication systems. Furthermore, the integration of quantum-resistant algorithms into hybrid systems is being explored to enhance the robustness of encryption against quantum-powered attacks.
Real-Time Threat Detection and Mitigation
Quantum computing’s unparalleled processing power opens up opportunities for real-time cybersecurity threat detection and mitigation. Quantum algorithms, such as Grover’s algorithm, enable faster analysis of large datasets, allowing organizations to identify and neutralize threats more effectively. For example, quantum-enhanced machine learning models can analyze network traffic patterns to detect anomalies indicative of cyberattacks.
A recent study by Cisco revealed that only 30% of companies globally are prepared to address AI-driven threats. However, the integration of quantum computing into AI systems could significantly improve this readiness by enabling faster and more accurate threat detection. Additionally, quantum-powered predictive analytics can help organizations anticipate potential vulnerabilities, allowing them to implement proactive measures to mitigate risks.
Revolutionizing Data Storage and Retrieval
Quantum computing offers transformative opportunities in data storage and retrieval by enabling the development of quantum memory systems. These systems leverage the principles of quantum superposition and entanglement to store and retrieve vast amounts of data more efficiently than classical systems. For instance, quantum memory can store multiple states simultaneously, significantly increasing storage capacity and retrieval speed.
This advancement has profound implications for industries that rely on large-scale data processing, such as healthcare, finance, and logistics. For example, quantum memory could enable faster analysis of genomic data for personalized medicine or optimize supply chain operations by processing real-time data more efficiently. Companies like IBM and Google are actively exploring the development of quantum memory systems to address these opportunities.
Advancing Artificial Intelligence in Cybersecurity
Quantum computing has the potential to revolutionize artificial intelligence (AI) applications in cybersecurity by enhancing the capabilities of machine learning algorithms. Quantum-enhanced AI can process and analyze complex datasets more efficiently, enabling the development of advanced cybersecurity tools. For example, quantum-powered AI models can improve the accuracy of intrusion detection systems by identifying subtle patterns in network traffic that classical systems might overlook.
Additionally, quantum computing can accelerate the training of machine learning models, reducing the time required to develop and deploy new cybersecurity solutions. This capability is particularly valuable in addressing emerging threats, such as AI-driven attacks and social engineering schemes. A recent report highlights how companies like Boeing are exploring quantum-powered AI applications to enhance safety and reliability in critical systems.
Strengthening Blockchain Technology
Quantum computing presents opportunities to strengthen blockchain technology by addressing its scalability and energy efficiency challenges. While quantum computers pose a threat to traditional blockchain encryption methods, they also offer solutions to enhance blockchain security and performance. For instance, quantum algorithms can optimize consensus mechanisms, reducing the time and energy required to validate transactions.
Moreover, quantum-resistant cryptographic techniques can be integrated into blockchain systems to safeguard them against quantum-powered attacks. This development is particularly important for industries that rely on blockchain for secure transactions, such as finance, supply chain management, and healthcare. Companies like Sectigo are actively researching quantum-resistant blockchain solutions to address these challenges.
Quantum-Powered Risk Assessment
Quantum computing enables more accurate and comprehensive risk assessment by processing complex datasets and simulations at unprecedented speeds. This capability is particularly valuable for industries that require precise risk modeling, such as insurance, finance, and cybersecurity. For example, quantum-powered simulations can analyze potential attack vectors and their impact on an organization’s infrastructure, allowing for more effective risk mitigation strategies.
A recent report highlights how quantum computing can enhance risk assessment in cybersecurity by identifying vulnerabilities in encryption methods and network defenses. Additionally, quantum-powered predictive analytics can help organizations anticipate future threats, enabling them to allocate resources more effectively to address potential risks.
Enhancing Secure Communication Networks
Quantum computing offers opportunities to develop secure communication networks that leverage quantum mechanics to ensure data confidentiality and integrity. For instance, quantum entanglement can be used to create secure communication channels that are immune to eavesdropping. This technology has significant implications for industries that require secure communication, such as government, defense, and finance.
A recent report highlights how quantum algorithms are being used to fortify communication networks against sophisticated cyber threats. Companies like Google and IBM are actively exploring the development of quantum-secure communication systems to address these challenges.
Facilitating Post-Quantum Transition
As quantum computing continues to advance, organizations must prepare for the transition to a post-quantum era by adopting quantum-resistant encryption methods and protocols. This transition presents opportunities for businesses to enhance their cybersecurity posture by implementing forward-thinking strategies. For example, companies can invest in quantum-resistant algorithms to protect sensitive data and develop training programs to improve employee awareness of quantum-related risks.
A recent Cisco report emphasizes the importance of proactive measures to address quantum-related challenges, highlighting the need for organizations to stay ahead of emerging threats. By adopting quantum-resistant solutions, businesses can ensure long-term data protection and resilience against quantum-powered attacks.
Conclusion
The advent of quantum computing heralds a new era in information security, characterized by both unprecedented challenges and transformative opportunities. As quantum computers inch closer to practical reality, the urgency to transition to quantum-safe cryptographic systems becomes paramount. The potential for quantum algorithms to break existing encryption methods poses a significant threat to data privacy and critical infrastructure, necessitating proactive measures to safeguard sensitive information (The Soufan Center).
Despite the hurdles in transitioning to quantum-resistant systems, such as interoperability issues and resource constraints, the benefits of embracing quantum technologies are substantial. Quantum-enhanced cryptography, real-time threat detection, and advancements in data storage and retrieval are just a few of the opportunities that quantum computing presents (SSL.com). Moreover, the integration of quantum computing with emerging technologies like artificial intelligence and blockchain promises to revolutionize cybersecurity, offering more robust defenses against increasingly sophisticated cyber threats.
As organizations and governments worldwide prepare for the quantum era, collaboration and investment in research and development are crucial. By fostering international cooperation and standardizing quantum-resistant technologies, stakeholders can ensure a coordinated response to the challenges posed by quantum computing. Ultimately, the successful navigation of this transition will determine the resilience of our digital infrastructure in the face of quantum-powered threats (Global Risk Institute, 2024).
References
- Quantum Computing Inc, 2023, Quantum Computing Inc
- KPMG Australia, 2024, KPMG Australia
- CISA, 2024, CISA
- GovLoop, 2024, GovLoop
- The Soufan Center, 2024, The Soufan Center
- SSL.com, 2024, SSL.com
- Global Risk Institute, 2024, Global Risk Institute